Some of the things we’re most interested in are described below. Our group has two main research areas β the physics of astrophysical plasmas, and the fluid dynamics of astrophysical dust and its interaction with gas. Plasmas are usually turbulent (chaotic), which is important for problems like generating magnetic fields (dynamos), cosmic-ray propagation, and the heating of the solar corona. We can make amazingly precise measurements of these processes in action using spacecraft in situ in the solar wind. Dust is important all over the place in astrophysics, since around half of the metals in galaxies are locked up in the solid (dust) phase. A particularly interesting application for us is to planetesimal formation, where fluid instabilities between dust and gas (the “streaming instability“) are thought to be key to clumping the dust so that it can eventually become self gravitating.
Kinetic plasmas and turbulence
Outside collaborators: Alex Schekochihin (Oxford), Matt Kunz (Princeton), Eliot Quataert (Princeton), Bill Dorland (Maryland), Chris Chen (Queen Mary), Lev Arzamasskiy (IAS), Trevor Bowen (Berkeley)
What’s the heat capacity of a hot plasma, like the solar wind or intracluster medium? Despite sounding simple, this question is actually rather difficult, because collisions are too infrequent to keep the system in local thermodynamic equilibrium. So, its heat capacity depends on which species you’re talking about (electron vs. ions, minor ions, nonthermal particles), and even on how your stir it up! The important processes are usually controlled by turbulence, which allows the largest motions to break down into smaller ones that can actually heat the particles efficiently.
In strongly magnetized plasmas (low π½), such as that being probed by Parker Solar Probe close to the sun, we found a surprising effect. If the turbulence is imbalanced (meaning that there are more waves in one direction than the other), the turbulent cascade, which usually makes smaller-scale motions, is “stopped” at the ion gyro scale. We called this effect the “helicity barrier”. It causes the turbulence to grow in time, rather than heating up the plasma! The effect explains the decades-long problem of the origin of the ion-scale kinetic transition (a steepening of the magnetic spectrum) observed in the solar wind, as well as suggesting lots of interesting implications for how it’s heated. Our work showing how such turbulence can drive strong ion heating through “ion cyclotron waves” was covered in various popular articles and podcasts (e.g., EOS, nature, ABC, CNET, and ODT)
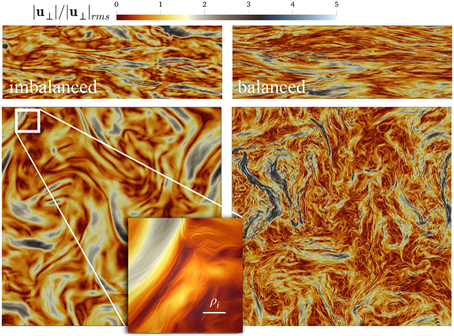
We also study the weakly magnetized case (high π½). A bizarre characteristic of these plasmas is that their fluid properties β how they move when perturbed on large scales β are controlled by micro-scale (ion gyro-scale) kinetic plasma instabilities like the mirror and firehose instabilities. We’re interested in understanding how these plasmas behave: how they become turbulent, how they generate magnetic fields, and how the small and large scales interact. They exhibit a variety of interesting effects that differ from MHD, related to the generation of pressure anisotropy (e.g., “interruption” and “magneto-immutability“) and the complicated nonlinear evolution of micro-scale instabilities.
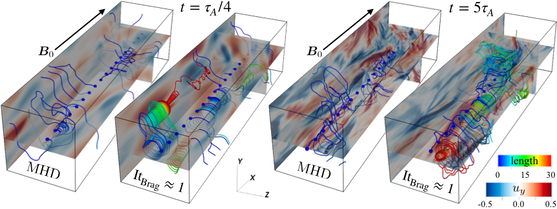
Solar wind switchbacks
Outside collaborators: Ben Chandran (UNH), Alfred Mallet (Berkeley), Stuart Bale (Berkeley)
Parker Solar Probe has been seeing an abundance of “switchbacks” β sudden reversals of the magnetic field inside the solar wind turbulence. There’s a great video from NASA here and an article that features our work here. We have been able to reproduce switchback magnetic-field structures in or simple expanding-box MHD simulations, starting from just simple superpositions of outwards-propagating AlfvΓ©n waves. This suggests that switchbacks originate in the wind itself as it propagates outwards (in situ) rather than bring remnants of solar-surface processes.
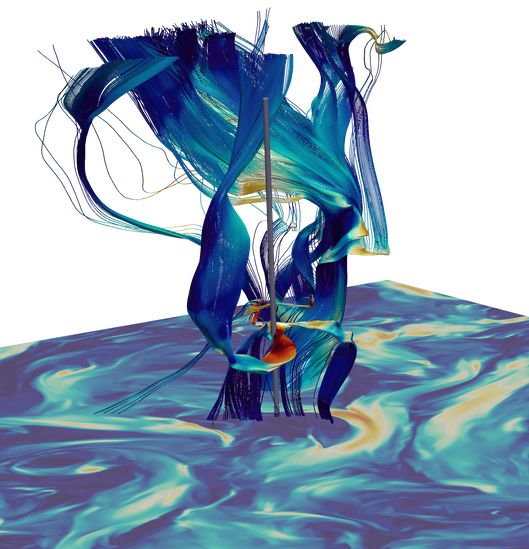
Astrophysical dust dynamics
Outside collaborators: Phil Hopkins (Caltech)
Many astrophysical fluids and plasmas are filled with macroscopic particles, or dust. The gas and the dust is often not perfectly coupled together, allowing for a variety of interesting dynamics. Working with Phil Hopkins, we showed that dusty fluids where the two phases are drifting with respect to one another are almost always unstable to the “Resonant Drag Instability” (RDI). The mathematics is quite neat β one can show that whenever the phase velocity of a wave in the fluid matches the difference in speed between the dust and the gas, an instability results (see our RDI letter). We discovered a variety of new instabilities using this technique, and it also helped in understanding the physics of the “streaming instability,” which is thought to be key for forming planetesimals in disks. How does the RDI-generated turbulence develop and saturate? What role does it play in the planet formation process? This work was featured in a Scientific American article.
fromΒ Seligman et al. (2018). As the RDI grows, the dust forms
into dense sheets and filaments before breaking into turbulence.
Movie credit: Phil Hopkins
Cosmic Rays
Outside collaborators: Phil Hopkins (Caltech), Eliot Quataert (Princeton), Philipp Kempskii (Berkeley)
Cosmic rays (CRs) are extremely high-energy relativistic particles that fly around the interstellar medium of our Milky Way and other galaxies. They are accelerated in supernovae and other extreme events, then propagate around the galaxy scattering off fluctuations in the magnetic field. The processes that regulate this propagation are not very well understood, but they must be reasonably efficient because we measure cosmic rays to be very isotropic here at Earth. We believe there are two main processes: self confinement, whereby CRs create magnetic fluctuations that then scatter themselves, and external turbulence, whereby CRs scatter from turbulence stirred up by other processes. But, in work with Phil Hopkins we showed that neither of these seem to work properly in explaining observations: the self confinement is too efficient, while the extrinsic turbulent is not efficient enough! To try and understand this, we have considered other processes that might change the effectiveness of the self confinement (e.g., charged dust), although it seems as though there are still important problems with current models. The impressive observations of CRs here on Earth provide very detailed constraints, but there’s lots left to understand!
Other interests
Some recent collaborators: Amitava Bhattacharjee (Princeton), Valentin Skoutnev (Princeton), Froncois Rincon (IRAP)
A great thing about studying astrophysics theory is how easy it is to explore other areas. My thesis studied the “large-scale dynamo” β how large-scale, well-organized magnetic fields are generated from turbulence β as well as the magneto-rotational instability. We have a few large-scale dynamo projects continuing now, and there are lots of interesting, unsolved problems.
Some other things β
- I’ve enjoyed working on Hamiltonian and Lagrangian mechanics. A particular interest is on symplectic integration, which involves designing numerical algorithms that respect the geometry of the underlying system. This generally leads to very nice algorithms with good conservation properties. For example, one can derive a complete particle-in-cell integration algorithm (for fields and particles) from a single Lagrangian.
- I like writing Mathematica code, in which you can solve all sorts of problems that would be very hard in a more tradiational language. The VEST package provides advanced tools for solving vector calculus problems (it simplifies enormous expressions, usually much better than you could do by hand), while NMSTools extends NDSolve to allow the numerical solution of eigenvalue ODEs.
Posters and talks β
Here are a few scientific talks and (older) posters. Feel free to copy images or videos, but please attribute the source.
KineticTurbulence.key ββ APS DPP talk about plasma heating
HighBetaPlasmas.key β a colloquium-style talk on high-Ξ² plasmas.
DustInstabilities.key β a colloquium-style on our RDI work and planet formation.
MagneticShearCurrent.key β half-hour talk about my thesis work.